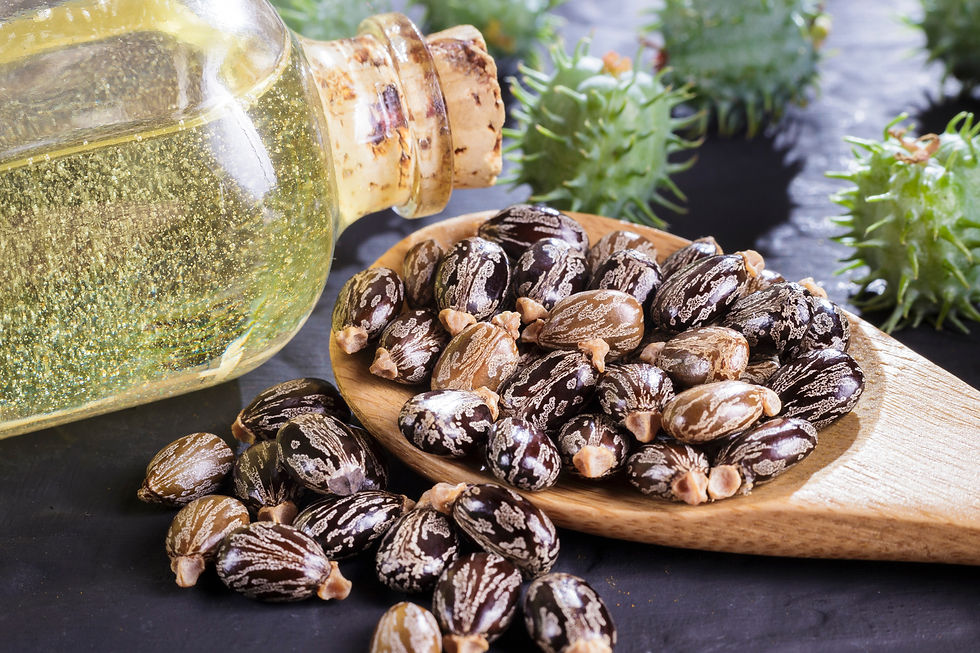
I decided to take a little break from writing about ketones, to look at other potential energy substrates for the brain. Also, previous posts have tended to be informative but long, crammed with facts and information which might prove difficult to digest for the casual reader. As such, I will attempt to ensure future posts are less technical and shorter, to make the content more accessible.
Now back to our topic….
The Summary - The process I intend to review is known as anaplerosis and the molecule of interest is Triheptanoin. The idea here is that many conditions, both neurological and metabolic, result from problems with cellular energy production. In many instances, abnormalities of the TCA cycle (the main source of cellular energy production) may be the culprit. As with ketones, anaplerotic molecules such as Triheptanoin can theoretically bypass metabolic blockages in the TCA cycle and improve cellular energetics. Whether this translates into improved clinical outcomes remains to be seen.
Ok, let’s dive right in ……
So what the heck is anaplerosis anyway?
Anaplerosis involves the replenishing of metabolic pathways by the addition of intermediates that have been extracted for metabolic functions. (Stay with me here – it will make more sense in a moment). The main target is the citric acid (TCA cycle). As the reader will recall, the TCA cycle is the series of chemical reactions used to generate energy (and other important cellular molecules) via the oxidation of acetyl-CoA derived from fats, proteins and sugars (see earlier post on Cerebral Energetics). During normal cellular functioning, components of the TCA cycle remain fairly constant. However, as many of these molecules are used in other cellular reactions, they may require replacing – a process known as anaplerosis or anaplerotic reactions. For example, metabolism of odd carbon chain fatty acids (you will recall fatty acids have a hydro-carbon backbone) can produce succinyl-CoA, an important constituent of the TCA cycle. From a practical point of view, anaplerotic metabolism takes place without most individuals being aware that it actually exists and is generally not associated with any disease state in the normal, healthy individual.
Below is a detailed map of the TCA cycle for your reference;
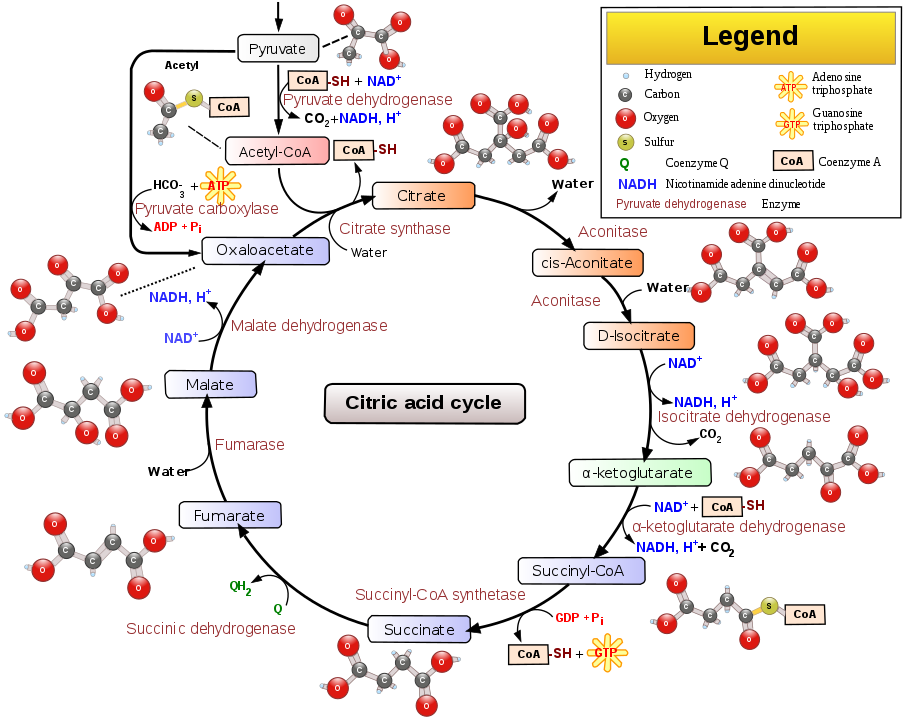
The tricarboxcylic acid cycle – also known as citric acid cycle or Krebs cycle
(By Narayanese, WikiUserPedia, YassineMrabet, TotoBaggins - https://commons.wikimedia.org/w/index.php?curid=6217701)
Here is a more condensed version of the TCA cycle showing how anaplerotic molecules are able to replenish TCA intermediates;

Simplified TCA cycle and anaplerosis in CNS and muscle. Red numbers indicate anaplerotic pathways, that can refill the levels of C4 intermediates of the cycle
(From – Mochel F, DeLonlay P, Touati G, et al. Pyruvate carboxylase deficiency: clinical and biochemical response to anaplerotic diet therapy. Mol Genet Metab. 2005;84(4):305-312. doi:10.1016/j.ymgme.2004.09.007 is marked with CC by 4.0)
Anaplerosis as a concept is interesting but so what?
While for most of us, the concept of anaplerosis is merely theoretical, this is not the case with individuals born with genetic disorders affecting the enzymes (an enzyme is a molecule that speeds up the rate of chemical reactions) used in these metabolic processes. The best described abnormalities are the long chain fatty acid oxidation disorders (LC-FAOD).
Aside – it is not my intent to go into these disorders in detail. My intent is to demonstrate the clinical consequences of disorders affecting normal cell metabolism as a precursor to other discussions
These disorders result from enzyme abnormalities leading to problems with transporting and oxidizing long chain fatty acids. This leads to problems with energy production, especially during fasting and physiological stress and impacts multiple organ systems. Patients are at risk of myopathies (disease of muscle) and neuropathies (disease of nerves), liver dysfunction, hypoglycaemia and cardiomyopathy (disease of the heart muscle). The disorder typically presents in the neonate but can develop later in life. Individuals are at constant risk of metabolic decompensation.
The treatment (until recently) was largely based on expert consensus and consisted of avoiding fasting, a diet rich in carbohydrates and low in long-chain fats and supplemented with medium chain triglyceride (MCT) oil and essential fatty acids. L-carnitine is sometimes prescribed. This regimen was only partially effective. It was not until the development of the anaplerotic molecule known as Triheptanoin that significant progress was made in managing these disorders.
What is Triheptanoin?
Triheptanoin is a triglyceride containing three odd carbon chain fatty acids (C7 or heptanoate). It was originally synthesized from caster bean oil and used in the food and cosmetic industry. The molecule is cleaved in the gut into heptanoate and glycerol and metabolised in the liver.

Chemical structure of triheptanoin
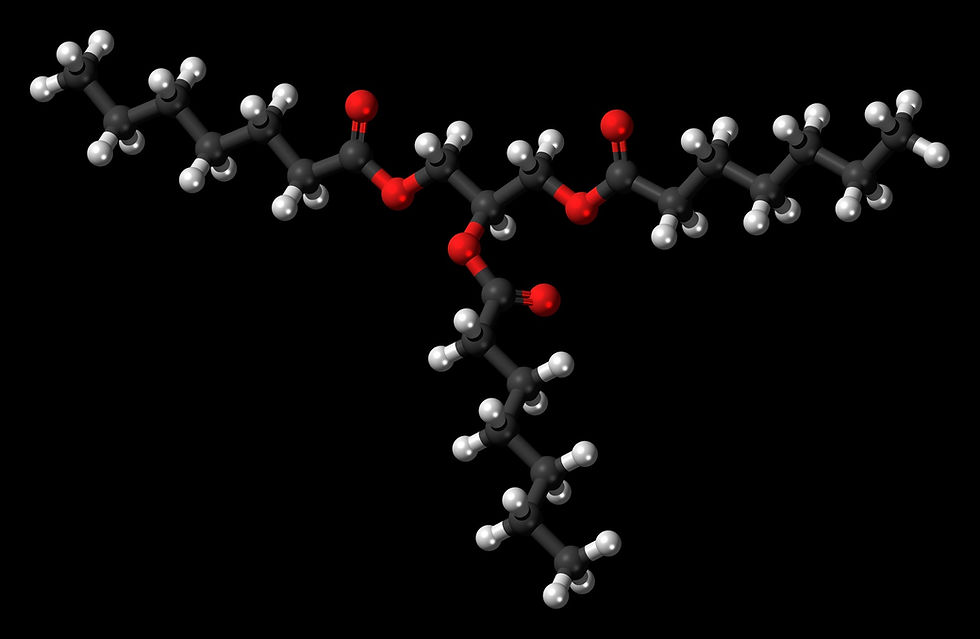
3D example of a molecule of triheptanoin
("File:Triheptanoin 3D ball.png" by Jynto (talk) is marked with CC0 1.0)
Upon entering the cell, heptanoic acid can be metabolised into a number of molecules.
The first is Acetyl-CoA, which as we know from cerebral energetics, can enter the TCA cycle to produce ATP.
Aside - CoA is derived from vitamin B – In its acetyl form is a very versatile molecule for both anabolic and catabolic pathways
The second are 5 carbon fatty acids which are able to generate five carbon ketone bodies (beta-ketopentanoate and beta-hydroxypentanoate) – which can enter the brain and may be neuroprotective
And lastly, propionyl-CoA may be produced which can be converted to succinyl-CoA, an important intermediate in the TCA cycle – thus becoming an anaplerotic reaction. Note – four carbon ketones such as acetoactetate and beta-hydroxybutyrate are also produced during this process.
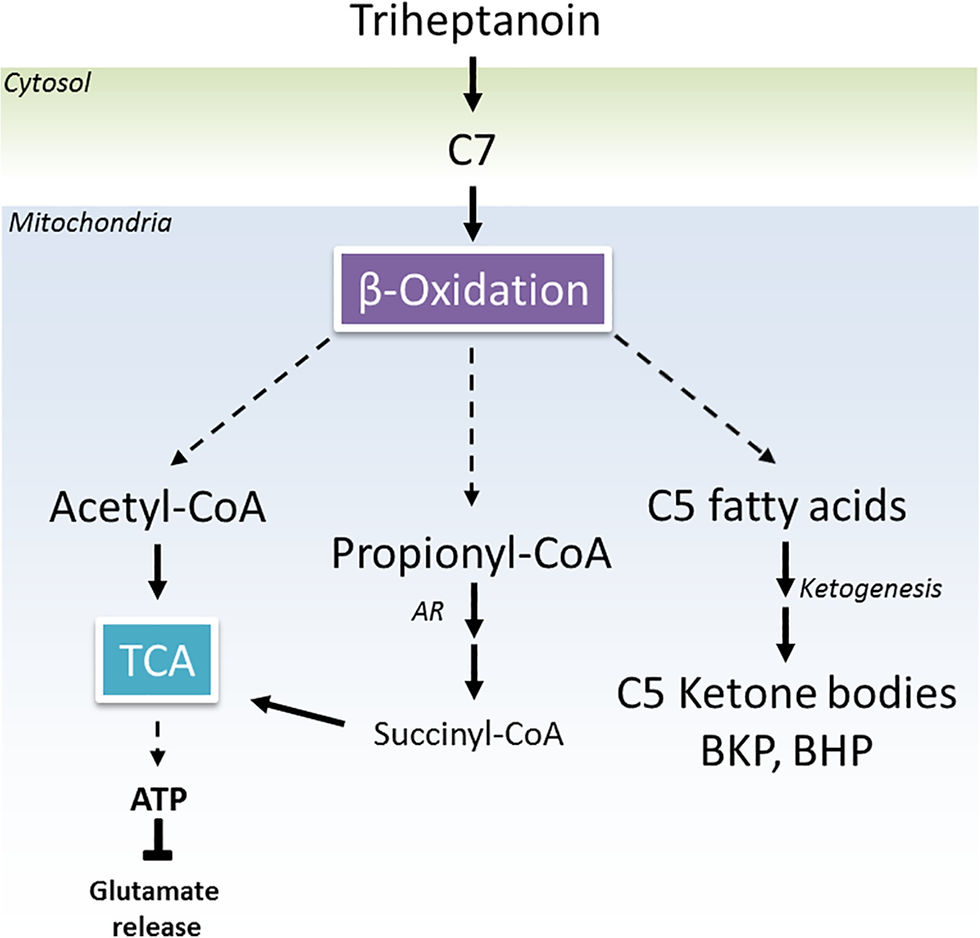
Schematic representation of the metabolism of triheptanoin
(Wehbe Z, Tucci S. Therapeutic potential of triheptanoin in metabolic and neurodegenerative diseases. Jrnl of Inher Metab Disea. 2020;43(3):385-391. doi:10.1002/jimd.12199)
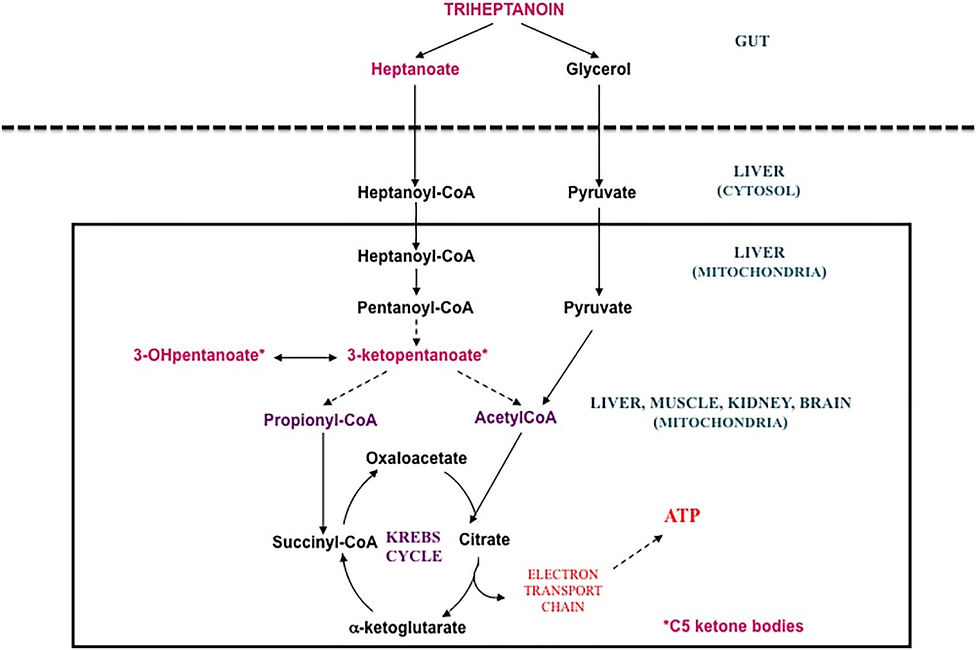
Metabolism of triheptanoin and the TCA.
(Mochel F. Triheptanoin for the treatment of brain energy deficit: A 14-year experience: Triheptanoin and Treatment of Brain Energy Deficit. Journal of Neuroscience Research. 2017;95(11):2236-2243. doi:10.1002/jnr.24111)
Ok, so are there any clinical applications?
The primary application for triheptanoin (and the only one for which its licensed) is in the treatment of paediatric and adult patients with molecularly confirmed LC-FAOD. This is based on the outcomes of a study by Vockley et al who administered trihepanoin (25-35% daily calorie intake) to 29 patients with LC-FAOD for 78 weeks. They found a 48% decrease in major clinical events such as hypoglycaemia, cardiomyopathy and rhabdomyolysis (muscle break down). Triheptanoin was well tolerated with gastro-intestinal upset the commonest side effect.
This is interesting as it was initially thought that MCT oil, which supplies acetyl-CoA groups to the TCA cycle, would be able to provide the necessary energy to avoid organ dysfunction. However, in clinical trials, MCT was found to be only partially effective. This led to the suggestion that the loss of TCA cycle intermediates (eg succinyl-CoA) may be responsible for the energy impairment – despite adequate acetyl-CoA. Therefore, only anaplerotic substances would have the potential to reverse the energy deficit.
Other studies (see Sklirou et al) have found similar outcomes with one reporting a mortality decrease from 65% on conventional diets to 3.8% on triheptanoin. (There are also a number of case series and case reports which can be found if references). Thus, anaplerotic molecules such as hepatanoate are capable of (at least partially) restoring TCA function and energy production.
Aside - With this background information, we can now turn our attention to the use of anaplerotic molecules to restore energy imbalances in the neurological diseases.
Can anaplerotic substances improve energetics in neurological disorders?
The most experience in using triheptanoin in neurological disorders is with epilepsy. Certain types of epilepsy are associated with impaired glucose metabolism leading to a lack of cerebral ATP. Furthermore, a deficiency of TCA intermediates in the brain may lead to hyper-excitability and seizures. One theory as to a possible mechanism is that the TCA intermediate alpha-ketoglutarate is a precursor for several neurotoxic neurotransmitters (eg glutamate). As production of glutamate is increase during seizure activity, this would lead to reduce levels of alpha-ketoglutarate and TCA cycle dysfunction.
Both heptanoate and C5 ketones can serve as an energy source for the brain. Studies in animals demonstrate hepatanoate can improve glucose utilization, replenish TCA cycle intermediates and provide antioxidant activity. Mice with seizure disorders fed triheptanoin were noted to have a significant decrease in seizure activity in low glucose conditions. Dr Karin Borges (who is arguably the worlds leading authority on triheptanoin use for epilepsy) conducted 2 studies examining the use of triheptanoin in humans. 10 adults were administered triheptanoin as add-on treatment (40-100 ml/d) for 27 – 513 days. Although only 2 finished entire 48 weeks treatment, 5/10 experienced > 50% reduction in focal seizures. The only adverse reactions were related to GI.
Aside - Clearly more research is necessary to determine triheptanoin has any clinical benefit in patients with epilepsy, but it is an interesting idea.
Trihepanoin has been used in the treatment of several other conditions. Although, very limited information and small studies but worth hearing about
Huntington’s disease is an inherited progressive neurodegenerative condition characterised by abnormal movements, psychiatric problems and dementia. No cure exists so management is largely supportive. Adanyeguh et al, using magnetic resonance spectroscopy (similar to MRI but used to study metabolic changes), demonstrated that patients with HD had an abnormal brain energy profile at baseline which significantly improved following a month of triheptanoin. (the study did not examine clinical outcomes)
Studies in animal models of ischemic stroke found that mice that were fed triheptanoin for 14 days prior to the onset of stroke, maintained cerebral glucose levels, had decrease cerebral neurotoxins and had smaller strokes then animals fed ordinary diet. The benefits appear to be due to an improvement of mitochondrial function and preservation of the cellular energy state.
Another study looked at the impact of triheptanoin on heart muscle function in a rat model of left ventricular hypertrophy (very thickened heart muscle). Seems that the diet reduced ventricular wall thickness and improved diastolic heart function compared to controls.
And finally, Comhair et al looked at the potential for anaplerotic substances to improve fatty liver disease in a mouse model of non-alchoholic fatty liver disease based on research in high protein diets. However, no benefit was found.
What’s the take home message?
While the concept of anaplerosis and replenishing TCA cycle intermediates appears scientifically plausible, the translation into clinical applications is largely limited to treatment of underlying metabolic disorders (such as long chain fatty acid oxidation disorder). Whether anaplerotic substances such as triheptanoin will prove to be beneficial in a broader range of neurological disorders (where energy deficits play a role) remains to be seen.
References
Adanyeguh IM, Rinaldi D, Henry P-G, et al. Triheptanoin improves brain energy metabolism in patients with Huntington disease. Neurology. 2015;84(5):490-495.
Augustin K, Khabbush A, Williams S, et al. Mechanisms of action for the medium-chain triglyceride ketogenic diet in neurological and metabolic disorders. The Lancet Neurology. 2018;17(1):84-93. doi:10.1016/S1474-4422(17)30408-8
Borges K, Kaul N, Germaine J, Kwan P, O’Brien TJ. Randomized trial of add‐on triheptanoin vs medium chain triglycerides in adults with refractory epilepsy. Epilepsia Open. 2019;4(1):153-163. doi:10.1002/epi4.12308
Borges K, Kaul N, Germaine J, Carrasco‐Pozo C, Kwan P, O’Brien TJ. Open‐label long‐term treatment of add‐on triheptanoin in adults with drug‐resistant epilepsy. Epilepsia Open. 2020;5(2):230-239. doi:10.1002/epi4.12391
Borges K, Sonnewald U. Triheptanoin—A medium chain triglyceride with odd chain fatty acids: A new anaplerotic anticonvulsant treatment? Epilepsy Research. 2012;100(3):239-244. doi:10.1016/j.eplepsyres.2011.05.023
Calvert S, Barwick K, Par M, Ni Tan K, Borges K. A pilot study of add-on oral triheptanoin treatment for children with medically refractory epilepsy. European Journal of Paediatric Neurology. 2018;22(6):1074-1080. doi:10.1016/j.ejpn.2018.07.014
Comhair TM, Garcia Caraballo SC, Dejong CHC, Lamers WH, Koehler SE. The odd-carbon medium-chain fatty triglyceride triheptanoin does not reduce hepatic steatosis. Clinical Nutrition. 2017;36(1):229-237. doi:10.1016/j.clnu.2015.11.005
Hartman AL. Neuroprotection in metabolism-based therapy. Epilepsy Research. 2012;100(3):286-294. doi:10.1016/j.eplepsyres.2011.04.016
Kim TH, Borges K, Petrou S, Reid CA. Triheptanoin reduces seizure susceptibility in a syndrome-specific mouse model of generalized epilepsy. Epilepsy Research. 2013;103(1):101-105. doi:10.1016/j.eplepsyres.2012.09.016
Mochel F. Triheptanoin for the treatment of brain energy deficit: A 14-year experience: Triheptanoin and Treatment of Brain Energy Deficit. Journal of Neuroscience Research. 2017;95(11):2236-2243. doi:10.1002/jnr.24111
Mochel F, DeLonlay P, Touati G, et al. Pyruvate carboxylase deficiency: clinical and biochemical response to anaplerotic diet therapy. Mol Genet Metab. 2005;84(4):305-312. doi:10.1016/j.ymgme.2004.09.007
Nguyen TD, Shingu Y, Amorim PA, Schwarzer M, Doenst T. Triheptanoin Alleviates Ventricular Hypertrophy and Improves Myocardial Glucose Oxidation in Rats With Pressure Overload. Journal of Cardiac Failure. 2015;21(11):906-915. doi:10.1016/j.cardfail.2015.07.009
Roe CR, Brunengraber H. Anaplerotic treatment of long-chain fat oxidation disorders with triheptanoin: Review of 15years Experience. Molecular Genetics and Metabolism. 2015;116(4):260-268. doi:10.1016/j.ymgme.2015.10.005
Schwarzkopf TM, Koch K, Klein J. Reduced severity of ischemic stroke and improvement of mitochondrial function after dietary treatment with the anaplerotic substance triheptanoin. Neuroscience. 2015;300:201-209. doi:10.1016/j.neuroscience.2015.05.014
Shirley M. Triheptanoin: First Approval. Drugs. 2020;80(15):1595-1600. doi:10.1007/s40265-020-01399-5
Sklirou E, Alodaib AN, Dobrowolski SF, Vockley J. Physiological Perspectives on the Use of Triheptanoin as Anaplerotic Therapy for Long Chain Fatty Acid Oxidation Disorders. Front Genet. 2021;11:598760. doi:10.3389/fgene.2020.598760
Tefera TW, Wong Y, Barkl-Luke ME, et al. Triheptanoin Protects Motor Neurons and Delays the Onset of Motor Symptoms in a Mouse Model of Amyotrophic Lateral Sclerosis. Kobeissy FH, ed. PLoS ONE. 2016;11(8):e0161816. doi:10.1371/journal.pone.0161816
Vockley J, Longo N, Madden M, et al. Dietary management and major clinical events in patients with long-chain fatty acid oxidation disorders enrolled in a phase 2 triheptanoin study. Clinical Nutrition ESPEN. Published online December 2020:S2405457720310950. doi:10.1016/j.clnesp.2020.11.018
Wehbe Z, Tucci S. Therapeutic potential of triheptanoin in metabolic and neurodegenerative diseases. Jrnl of Inher Metab Disea. 2020;43(3):385-391. doi:10.1002/jimd.12199
Willis S, Stoll J, Sweetman L, Borges K. Anticonvulsant effects of a triheptanoin diet in two mouse chronic seizure models. Neurobiology of Disease. 2010;40(3):565-572. doi:10.1016/j.nbd.2010.07.017
Comments